Species management advice
Here, we provide genetic knowledge of some marine species in the Baltic Sea. Based on our studies, we discuss species potential to adapt to new environmental conditions, and highlight threats to the genetic diversity. We also present some advice for informed management of species and Baltic Sea biodiversity.
Baltic isopod
Baltic isopod, Idotea balthica, is a key species in shallow-water Baltic Sea habitats. It is an important food source for coastal fish, and also the most important grazer on Fucus bladder wrack and narrow wrack). Sometimes this isopod becomes extremely abundant, resulting in the complete removal of Fucus in that area.
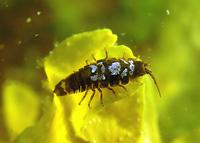
Contact
Pierre DeWit, Department of Marine Sciences – Tjärnö
Bladder wrack
Bladder wrack, Fucus vesiculosus, is the most dominant and ecologically important macro alga in the Baltic Sea. It provides essential ecosystem services as maintainers of local biodiversity, but also as nurseries for commercially important fish species. Recent genetic data show that bladder wrack and narrow wrack (see below) are very closely related. In several cases, analyses fail to separate them from each other. Ecologically, they both provide important ecosystem functions in the Baltic Sea particularly as habitat forming organisms.
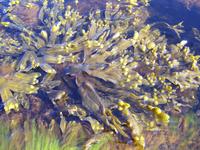
Contact
Kerstin Johannesson, Department of Marine Sciences – Tjärnö
Ricardo Pereyra, Department of Marine Sciences – Tjärnö
Brown trout
Brown trout, Salmo trutta, is an ecologically and culturally important species in many freshwater habitats. It is a host of the threatened freshwater pearl mussle Margaritifera margaritifera.
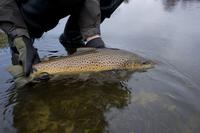
Contact
Linda Laikre, Department of Zoology, University of Stockholm
Lovisa Wennerström, Department of Zoology, University of Stockholm
Baltic cod
Baltic cod, Gadus morhua, is one of the major cod stocks globally. It has a key role in the Baltic Sea with impact on the whole ecosystem, from the main pelagic fish herring to the zooplankton and phytoplankton.
Analyses of ancient cod DNA indicate that the eastern cod population has been isolated for a long time, possibly already from the time of the Baltic colonisation 6 000 to 8 000 years BP.
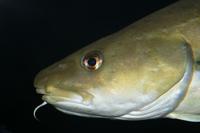
Contact
Carl Andre, Department of Marine Scieces – Tjärnö
Herring
The Baltic Sea herring, Cluepa harengus, is an important prey species for many predators including cod and seals. The species includes populations of autumn-spawning herrings, mainly in the western and southern Baltic Sea; and spring-spawning herring, mainly in the eastern and northern parts of the sea basin. There is wide overlap in the distribution of the different forms.
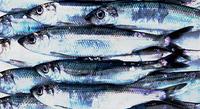
Contact
Linda Laikre, Department of Zoology, University of Stockholm
Narrow wrack
Narrow wrack, Fucus radicans, formed less than 8 000 years ago from bladder wrack inside the Baltic Sea. It has since spread and established along the coasts of the Bothnian Sea, and along the coast of Estonia in the Baltic Proper. Narrow wrack and bladder wrack are very closely related and are difficult to separate. Work is ongoing to resolve their taxonomic relationship. Ecologically, they both provide important ecosystem functions in the Baltic Sea particularly as habitat forming organisms.
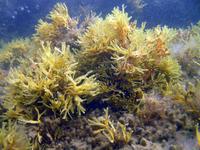
Contact
Kerstin Johannesson, Department of Marine Sciences – Tjärnö
Ricardo Pereyra, Department of Marine Sciences – Tjärnö
Nine-spined stickleback
The nine-spined stickleback, Pungitius pungitius, and the three-spined stickleback are taxonomically related and have a similar ecology. The two species can occur sympatrically in the Baltic Sea. However, the nine-spined seems to be less common in marine and brackish habitats than the three-spined stickleback. Also, the levels of genetic differentiation and diversity of the nine-spine sticklebacks differ from that of the three-spined sticklebacks.
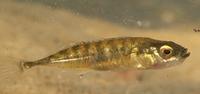
Contact
Britta Meyer, Max Planck Insitute for Evolutionary Biology, Germany
Pike
Pike, Esox lucius, is one of several freshwater fishes inhabiting the Baltic Sea. The species naturally prefer the less saline areas and thus colonize mostly the coastal areas. The pike is subject to commercial and sport fishery in coastal areas all around the Baltic. Data is currently missing on whether this fishery is affecting the genetic diversity of the species.
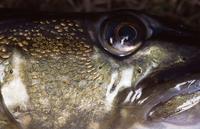
Contact
Lovisa Wennerström, Department of Zoology, University of Stockholm
Linda Laikre, Department of Zoology, University of Stockholm
Nils Ryman, Department of Zoology, University of Stockholm
Baltic salmon
The salmon, Salmo salar, reproduces in rivers across the whole Baltic Sea. Juveniles stay in freshwater streams for one to four years and then spend one to several years at sea on a feeding migration before they return to spawn in their natal river. The population structure of Baltic salmon has been known since the mid 1980s. Already then it was clear that the species should be managed on a population by population basis, and that releases were potentially harmful to native gene pools.
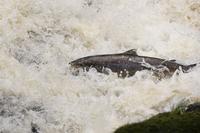
Contact
Linda Laikre, Department of Zoology, University of Stockholm
Sara Kurland, Department of Zoology, University of Stockholm
Three-spined stickleback
Three-spined stickleback, Gasterosteus aculeatus, is one of the most abundant fish species in the Baltic Sea, after herring and sprat. The stickleback has an influential position in the ecosystem as food source for piscivorous fishes and is an important predator on grazers, e.g. the Baltic isopod.
During spring spawning, high densities of sticklebacks migrate from offshore into shallow bays and harbors along the coast. Here, they provide a rich food source for adult stages of piscivorous fishes. The sticklebacks themselves also predate on a diverse range of prey items, including e.g. eggs and larvae of coastal piscivorous as well as mesograzers. Thus they affect recruitment on commercial fish and potentially also algal growth.
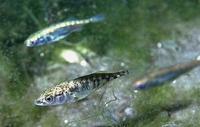
Contact
Britta Meyer, Max Planck Insitute for Evolutionary Biology, Germany
Melanie Heckwolf, GEOMAR Helmholtz centre for ocean research, Germany